E. M. Garcia Vivant
Listeria monocytogenes is the causative agent of the food-borne life threatening disease listeriosis. This pathogenic bacterium received much attention in the endeavor of deciphering the cellular mechanisms that underlie the onset of infection and its ability to adapt to the food processing environment. Although information is available on the presence of L. Monocytogenes in many environmental niches including soil, water, plants, foodstuff and animals, understanding the ecology of L.
Monocytogenes in outdoor environments has received less attention. Soil is an environmental niche of pivotal importance in the transmission of this bacterium to plants and animals. Soil composition, microbial communities and macrofauna are extrinsic edaphic factors that direct the fate of L. Monocytogenes in the soil environment. Moreover, farming practices may further affect its incidence. The genome of L.
Monocytogenes presents an extensive repertoire of genes encoding transport proteins and regulators, a characteristic of the genome of ubiquitous bacteria. Postgenomic analyses bring new insights in the process of soil adaptation. In the present paper focussing on soil, we review these extrinsic and intrinsic factors that drive environmental adaptation of L.
Fusion FzaPP Garden of Ku GATO Gawk GCD Gentleman GizzA Glam Affair Glitterati glow Gola Goucci grasp Grixdale Grumble Gypsys H.E.D. Hairfair Half Deer Happy Finds Happy Pencil Havok Hermony Heroes Art. Hmaem Hod HooT HouseofFox Ibizarre ieQED IKON illmatic Illusory imbue Imeka In her shoes india Indie Rose Ingenue inMonster insanya.
IntroductionCirculation of zoonotic and Human pathogens within the biosphere is a major health issue. Agroecosystems may participate to the transmission of pathogens to the food chain through production of contaminated raw products. However, as illustrated in Figure, understanding conditions that will trigger such contaminations or, on the opposite that will limit risks of contamination is difficult in the face of the complexity of the ecology of microorganisms. Listeria monocytogenes is the agent of listeriosis, a food-borne illness. Health effects range from flu-like symptoms with vomiting and diarrhea in healthy adults to life-threatening diseases such as meningitis and septicaemia in vulnerable people and spontaneous abortion in pregnant women.
In the light of these health hazards, this bacterium received much attention in order to understand the physiopathology of listeriosis. As a matter of fact, L. Monocytogenes has become a paradigm for the study of intracellular pathogens. Similarly, the mechanisms that underlie its ability to persist in foodstuff and in the food manufacturing environment have been documented. However, the ecology of L. Monocytogenes in outdoor environments is only partially understood. The objective of this review is to present the state of the art regarding extrinsic and intrinsic factors that shape the ecology of L.
Monocytogenes in the soil environment. Data available on the occurrence of L. Monocytogenes in soilSeveral reports on the incidence of L. Monocytogenes in soil are available (Table ). The pioneering work by Welshimer presented the first evidence that soil is an environmental niche for L. Monocytogenes and occurrence of the bacterium was observed in a third of the 12 sampled farms (Welshimer,; Welshimer and Donker-Voet, ).
This was further supported in the seventies by the work of Weis and Seeliger. These authors surveyed the occurrence of L.
Monocytogenes in 746 soil samples collected in Southern Germany. A total of 160 strains were isolated and the overall incidence of L. Monocytogenes was 21.4% (Weis and Seeliger, ). In this study, variations from 8.4 to 44.0 in the percentage of positive samples were observed according to the type of culture in the field and the highest incidence was recorded for uncultivated fields and meadows. Higher prevalence in meadows and uncultivated fields (30.8%) compared to cultivated fields (8.3%) was confirmed later (Dowe et al., ).
Similarly, in a recent survey of the distribution of the Listeria species in urban and natural environments in the US, while overall prevalence in soil was 19% in natural environments and 30% in urban environments, spatial variation of the prevalence of Listeria sp. Was observed within each category (Sauders et al., ). Temporal variation of the detection of L. Monocytogenes has been documented after sampling at the same site (Weis and Seeliger,; Sauders et al., ). Moreover, prevalence differed significantly according to the season and category of environment.
Indeed, it was highest during summer in natural environments but lowest at this time of the year in urban environments (Sauders et al., ). In another report of a 3-year survey on fruit and vegetable farms, prevalence was higher in winter except during 1 year (Strawn et al., ) and correlation between season and occurrence is not a clear-cut. Incidence of L. Monocytogenes in soil samples collected from small ruminant and cattle farms has also been recorded (Garcia et al.,; Nightingale et al.,; Fox et al., ).Listeria enrichment broth.In these surveys, detection always required selective enrichment. When available, the actual concentration of L. Monocytogenes reported from soil samples is low (MacGowan et al.,; Dowe et al., ). It suggests that the population of L.
Monocytogenes in soil is generally low. This was confirmed in a French nationwide survey where a PCR assay was performed on a collection of 1232 soil DNA for the specific detection of L. Monocytogenes (Locatelli et al., ). All samples were below the detection limit of 10 4 g −1 of dry soil.
Interestingly, a comparison of cultivation-based and molecular detection on a subset of 53 fresh soil samples showed that the incidence of culturable L. Monocytogenes was 17% but only one of these samples was positive for the molecular detection of L. Monocytogenes and its population was quantified at 2.88 10 4 g −1 of dry soil (Locatelli et al., ).These surveys clearly demonstrate that soil is an environmental niche of L. Monocytogenes but its population is generally low. Temporal and spatial variations of its occurrence point out that environmental factors drive the fate of L. Monocytogenes in soil. Edaphic factors that affect its survivalSoil is an extremely complex and heterogeneous environment composed of minerals, organic matter, lacy plant roots and a complex biota including microorganisms, viruses, mesofauna and macrofauna.
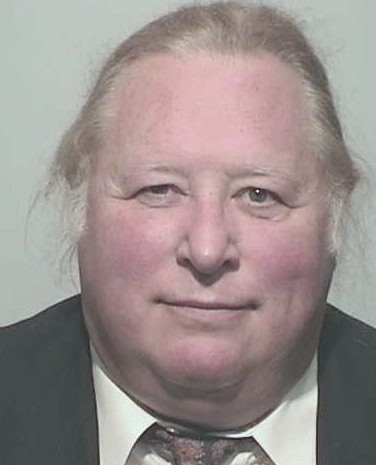
All of these are constantly interacting and soil may be regarded as an environment under dynamic equilibrium (Kogel-Knabner et al., ). Soil is characterized by complex food webs influenced by below-ground and above-ground processes (Wardle, ). As such, deciphering environmental drivers that impact the occurrence of L. Monocytogenes in soil is extremely hard as these drivers are interconnected.One of the conclusions that can be drawn from the results of the surveys presented above is that the nature of the soil affects the occurrence of L.
However, in these reports, indications of the soil characteristics are coarse. This makes it difficult to extract significant information regarding the relation between soil's characteristics and the presence of L.
Monocytogenes.Investigations on listerial population dynamics after inoculation of soil microcosms (Table ) evidenced that water availability is critical to its survival (Welshimer,; Picardbonnaud et al.,; Vanrenterghem et al.,; Dowe et al., ). The type of soil affects population dynamics. Sandy soil represents an environment less favorable for L. Monocytogenes than sandy loam and clay loam soils (Dowe et al., ); lower survival in clay soil than in fertile garden soil was also reported (Welshimer, ).
A drawback of these studies is that only one soil of each type was used. A statistical approach to the analysis of L. Monocytogenes survival in soils was recently performed to circumvent this limitation of the published data (Locatelli et al., ).
Data on the survival of L. Monocytogenes in 100 soil microcosms was analyzed in the light of a comprehensive and detailed characterization of the soil samples (Locatelli et al., ). These results confirmed that survival is dependent of the type of soil. Survival up to 84 days was observed in 71% of the soils tested while survival did not exceed 14 days in the rest of the soil microcosms.
Variance partitioning explained this short-term survival by the soil chemical properties, especially the basic cation saturation ratio. The long-term survival could be related to the soil texture and especially clay content. The report by Locatelli et al. And others evidenced that the pH of the soil is a major driver of the fate of L. Monocytogenes in soil (Weis and Seeliger,; Sidorenko et al.,; McLaughlin et al.,; Locatelli et al., ). Role of soil microflora, microfauna, mesofauna and macrofaunaSoil is a rich and complex biotic environment that generates massive interest because of the immense diversity it harbors and the many services associated (Nannipieri et al.,; Decaens,; Griffiths and Philippot, ).
E. M. Garcia Vivant La
The impact of indigenous microorganisms on the fate of L. Monocytogenes in soil has been addressed experimentally in microcosms. In these experiments, population dynamics of L. Monocytogenes were compared in autoclaved and non-autoclaved soil microcosms. The results clearly demonstrated that autoclaving facilitates implantation of L. Monocytogenes in soil as growth was observed in autoclaved soil while the population decreased in non-autoclaved soil (Welshimer,; Botzler et al.,; Vanrenterghem et al.,; Dowe et al.,; McLaughlin et al., ). However, this effect of the soil microflora cannot be observed in soils with low pH as a decline of the listerial population was observed even in sterilized soils (Locatelli et al., ).
Interestingly, this antagonistic effect depends on the diversity and the structure of the soil microflora (Vivant et al., ). In this report, experimental erosion of diversity clearly showed that loss of biotic diversity resulted in a better survival of L. Monocytogenes in soil.In the complex food web of the soil environment, interactions of L. Monocytogenes with microfauna, mesofauna and macrofauna are most likely and this assumption is supported by lab experiments.Interaction of L. Monocytogenes with bacteriophagous protozoans has been documented (Ly and Muller,; Barker and Brown,; Harf,; Gourabathini et al., ). Once internalized by endocytosis, it can grow within the cytoplasm of Acanthamoeba sp. And Tetrahymena pyriformis and eventually causes lysis and death of the host within 8–14 days (Barker and Brown,; Harf, ).
In a study focused on Glaucoma sp. Isolated from lettuce, Tetrahymena sp.
Isolated from spinach and Tetrahymena sp. Isolated from soil, intracellular survival of L. Monocytogenes was confirmed (Gourabathini et al., ).
Monocytogenes survives endocytosis by Acanthamoeba castellanii; this protozoan could actually enhance growth of the bacterium while feeding on various species of Listeria was not lethal to the protozoan (Zhou et al., ). These are indirect indications that protozoans can act as vectors of dispersion of L. Monocytogenes in soil. However, other reports suggest that the ability of L.
Monocytogenes to survive after ingestion by protozoans may be strain and/or species specific as killing of L. Monocytogenes by Acanthamoeba polyphaga, Acanthamoeba castellanii and Acanthamoeba lenticulata has been reported (Akya et al., ).Reports on the role of free living nematodes feeding on bacteria as vectors of dispersion of food-borne pathogens are available in the literature (Anderson et al.,; Caldwell et al.,; Gibbs et al.,; Thomsen et al.,; Forrester et al.,; Zhou et al.,; Guha et al., ). Ceanorhabditis elegans can feed on L.
Monocytogenes, moreover, in an agar plate assay, presence of live cells in the gut and excrement was confirmed and shedding of the pathogen was evidenced (Anderson et al.,; Caldwell et al.,; Guha et al., ). Interestingly, shedding by Diploscapter sp., a bacteriovorous soil nematode, was observed in soil amended with composted turkey manure (Gibbs et al., ). Feeding on L. Monocytogenes may have deleterious effects on C. Elegans (Forrester et al., ) and killing of the nematode was demonstrated experimentally (Thomsen et al., ) but others did not report killing (Guha et al., ) and this remains an open question.Information on interactions between L.
Monocytogenes and soil mesofauna and macrofauna is scarce (Kuzina et al.,; Mansfield et al.,; Sezen et al.,; Lapanje et al., ). However, these reports support the idea that several species of the soil meso- and macrofauna could act as reservoirs and vectors.
Indeed, Listeria sp. Was detected in the gut of the isopod Porcelio scaber (Lapanje et al., ) and in the gut of the Diptera Anastrepha ludens (Kuzina et al., ). Infection of another Diptera, Drosophila melanogaster, by L.
Monocytogenes has been demonstrated experimentally (Mansfield et al., ). In this work, intra-host multiplication was observed resulting in killing of the infected fruit flies within 6–8 days. Has been isolated from the bacterial flora of the Coleoptera Agelastica alni with a frequency of 18% (Sezen et al., ).
Finally, Listeria sp. Was detected in half of 10 wormfarms sampled but L. Monocytogenes was not isolated in any of them (Smith, ). Considering the diversity of the soil fauna (Decaens, ), one can assume that many species probably interact with L. Elucidation of these complex interactions in the soil food web would bring a new understanding of the ecology of this food-borne pathogen. Moreover, wild animals including mammals and birds must be considered as potential zoonotic reservoirs of the pathogen (Weis and Seeliger,; Yoshida et al.,; Lyautey et al., ) and may participate to its transfer to soil.
Farming practices and transfer into the soil (compost, sewage sludges, reclaimed waste)Telluric occurrence of L. Monocytogenes and other food-borne pathogens raises health issues in cultivated fields and grazing pastures as soil may be a vector of pathogens to cultivated plants and farmed animals. Hence, cattle and small ruminants are reservoirs of L. Monocytogenes (Garcia et al.,; Nightingale et al.,; Fox et al., ). There is a body of evidence that suggests that farming practices can directly impact on the circulation and implantation of L. Monocytogenes.Ensilage of contaminated crops may result in the increase of L. Monocytogenes populations in the final feed that may become the vector of cattle listeriosis (Fenlon,; Wiedmann et al., ).Agricultural recycling of organic wastes without sanitation procedures is a route of transmission of L.
Monocytogenes to soil. Indeed, stored sewage sludges frequently contain L.
Monocytogenes at low levels (1–240 bacteria MPN g −1 dry matter) and their agricultural use as fertilizer without sanitation procedure may facilitate its transfer and implantation into soil (Watkins and Sleath,; Alghazali and Alazawi,; De Luca et al.,; Garrec et al., ). Per hectare, spreading of one to two tons of sludge would bring around 10 6–10 8 L.
Monocytogenes per year (Garrec et al., ).The presence of L. Monocytogenes in the faeces of farm animals has been recognized (Fenlon et al.,; Nicholson et al.,; Nightingale et al., ). In a case-control study involving a total of 52 bovine, goat and sheep farms, the frequency of detection varied from 22 to 33% in bovine farms and from 3 to 18% in goat and sheep farms (Nightingale et al., ). Survival during storage of faecal wastes is limited to a few weeks but daily inputs in the storage facilities of the farmyard could maintain a constant load of viable L. Monocytogenes (Vanrenterghem et al.,; Hutchison et al., ).
Land spreading of these untreated wastes can contribute to the transmission of L. Monocytogenes to soil. In an experiment of spreading of artificially contaminated faecal wastes on a grass pasture, 64–128 days were required until the listerial population declined to undetectable levels (Hutchison et al., ). In a separate experiment maximum survival period varied from 4 days to over 32 days after land application according to the type of waste (Nicholson et al., ).
Delaying incorporation of faecal waste after land application on agricultural soils could result in a decline in the population of the pathogen (Hutchison et al., ). Under laboratory conditions, survival in bovine manure-amended soil varied from 21 to 43 days and depended on the dose and on the temperature of incubation (Jiang et al., ).Survival up to 56 days was observed in a climatic chamber experiment designed to investigate the fate of several pathogens after spreading of anaerobic digestion residues on grass crops (Johansson et al., ).Statistical integration of survey data gives interesting insights into the environmental parameters affecting the probability of occurrence of the pathogen.
Toyota dvd navigation system. Ivanek et al. Found a strong association between weather, soil properties and the probability to detect Listeria sp. In soil (Ivanek et al., ). In a recent 2 year long longitudinal study of the occurrence of food-borne pathogens in 5 fruit and vegetable farms, landscape and meteorological factors were associated to the frequency of positive samples (Strawn et al., ). Overall prevalence of L. Monocytogenes was 15% while in soil, the frequency of detection was 9%.
This survey was exploited to develop a classification tree model. This evidenced that temperature, proximity to surface water, roads/urban development and pasture/hay grass, but also soil-related parameters (available water storage, soil organic matter) were environmental and topographic factors of importance to explain detection of L. Monocytogenes (Strawn et al., ). Interestingly, in this survey, the frequency of detection of L. Monocytogenes was highest in water samples. The quality of water used for land irrigation is of concern and transfer of pathogens through poor quality irrigation water is likely (Steele and Odumeru,; Selma et al.,; Ijabadeniyi et al.,; Oliveira et al., ).
Proximity to dairy farms is an environmental factor that influences detection of L. Monocytogenes in watersheds (Sauders et al.,; Lyautey et al., ). Wastewater treated effluents frequently carry L. Monocytogenes (Watkins and Sleath,; Alghazali and Alazawi,; Steele and Odumeru,; Paillard et al.,; Odjadjare et al.,; Moreno et al., ) with loads varying from 3 to 15 CFU.ml −1 (Alghazali and Alazawi, ) to over 10 3 CFU.ml −1 (Odjadjare et al., ).
E. M. Garcia Vivant Rose
Intrinsic factors in relation to the gene content/transcriptional regulationSequencing of the genome of L. Monocytogenes yields insights into traits that permit persistence of the pathogen into soil. The description of the genome of L. Monocytogenes EGD-e, the first sequenced strain, highlighted features relevant to the ubiquity of this species (Glaser et al., ).
Indeed, genes encoding transport proteins represent a high proportion of the genome (11.6% of the genome of L. Monocytogenes EGD-e) 26% of them are related to the transport of carbohydrates by phosphoenolpyruvate-dependent phosphotransferase systems. It is thus equipped to have access to multiple carbon sources from varying environments. The proportion of regulatory genes is also high (7.3%).
Such an extensive repertoire of regulatory genes is expected from a versatile bacterium that thrives in many habitats. The role of some of them has been studied extensively, especially PrfA and VirR because of their importance during infection of the mammal host.At the moment, over 20 genomes of representatives of the genus Listeria are available (4 non L. Monocytogenes and 24 L.
Monocytogenes). These are circular chromosomes ranging from 2.7 to 3.0 Mb in length. A characteristic of these genomes is their high degree of synteny (den Bakker et al., ). These comparative genomic analyses identified around 4400 genes in the pan-genome of L. Among the putative protein coding genes (between 2330 and 2465 genes) about 80% are within the core genome.
This rather high proportion of core genes relative to several other species is consistent with a stable species backbone and a limited accessory genome (from 323 to 753 genes per strain) (den Bakker et al., ). Interestingly, genes involved in transport and metabolic processes are overrepresented in both core and accessory genomes (den Bakker et al., ). In silico comparison of the representation of Clusters of Orthologous Groups within the genomes of the genus Listeria, Bacillus, Lactobacillus, Staphylococcus and Streptococcus (Markowitz et al., ) illustrates the richness of all listerial genomes, similar to Bacillus genomes, in genes coding transport proteins and transcriptional regulators (Table ).
E. M. Garcia Vivant En
This could facilitate adaptation to various habitats, a trademark of the bacteria of the genus Bacillus and Listeria. Within the genus Listeria, the proportion of genes coding transport proteins, histidine kinases and transcriptional regulators are similar (Table ). Noteworthy is the overrepresentation of PTS systems in both core and accessory genomes (Glaser et al.,; Doumith et al.,; Deng et al.,; den Bakker et al., ). Such a pool of carbohydrate transporters and the ability to metabolize an extended range of these carbon sources could represent a fitness advantage under the control of selective pressure in the environment and in vivo (den Bakker et al., ). Under biotic conditions, the diversity of PTS systems and their possible functional redundancy could attenuate the efficacy of PTS-triggering bacteriocins (Diep et al.,; den Bakker et al., ) produced by neighboring bacteria (den Bakker et al., ). FunctionDistribution in taxonomic unitFirmicutesListeriaBacillusLactobacillusStaphylococcusStreptococcusN = 521N = 28N = 70N = 48N = 50N = 90Transport257 (204)320 (311)466 (466)208 (208)266 (266)216 (247)Carbohydrate transport20 (7)23 (23)32 (27)13 (21)7 (7)17 (10)Amino acid transport43 (44)40 (39)80 (63)48 (37)44 (44)41 (36)PTS27 (24)86 (86)29 (29)28 (5)25 (25)41 (41)Histidine kinase15 (9)15 (15)50 (35)8 (6)15 (15)9 (9)Transcriptional regulator89 (65)142 (144)204 (170)70 (70)77 (55)68 (61). FunctionFirmicutesBacillus subtilisListeria sp.L.